Fiber Optic Reflection/Backscatter Probe Bundles with Reference Leg
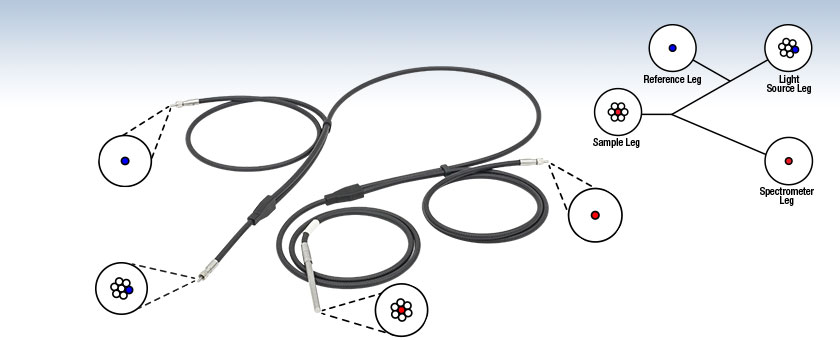
- Fiber Y-Bundles for Reflection and Backscatter Spectroscopy
- 4 Legs: Source, Reference, Sample, and Signal
- Ø200 µm Core High- or Low-OH Multimode Fiber
RP26
Reflection Probe with Ø1/4"
Probe Sample Leg to SMA
Connectors and Reference
Spectrometer Leg
Reference Spectrometer
End
Light Source End
Sample End
Spectrometer
End

Please Wait
Typical Reflection Spectroscopy Setup
Click to Enlarge
Typical reflection spectroscopy setup using Thorlabs' reflection probe with reference arm, reflection probe holder, two CMOS spectrometers, and broadband fiber-coupled light source. Note: The positioning of the reference fiber in the light source end is not guaranteed to match the schematic.
Features
- Fiber Optic Y-Cables with Reference Leg for Reflection and Fluorescence Spectroscopy
- Light Source End Illuminates Sample
- Sample Leg Equipped with a Ø1/4" Probe
- Spectrometer End Carries Reflected Light from the Sample
- Reference Leg Allows for Source Monitoring by Second Spectrometer
- Reinforced Stainless Steel Tubing and Steel Strain Relief Sleeves for Durability
- Both Y-Joints have Adjustable Fiber Clamps
- Legs with SMA Connectors Engraved with Fiber Orientation (See Specs Tab for Details)
Thorlabs' Fiber Bundle Probes with Reference Legs are optimized for measuring diffuse and specular reflectance, color, fluorescence, and backscattering of solid, liquid, and powder samples. They are bifurcated fiber cables with a leg to carry light from a source to a sample, a leg to carry light reflected by the sample to a spectrometer, and a reference leg to carry light from a source directly to a second spectrometer. Each SMA-terminated leg is engraved with the fiber orientation, and the reference leg is also engraved with an R to aid in identification (see the Specs tab for details). These probe bundles are ideal for use in applications where fluctuations in the source have a negative impact on spectral data. Each fiber bundle is built using Thorlabs' Ø200 µm core multimode fiber with either a high or a low hydroxyl ion (OH) content for use in the 250 - 1200 nm or 400 - 2400 nm range, respectively.
These fiber bundles each have a sample leg with a Ø1/4" (Ø6.35 mm) probe for manual applications. They have light source, spectrometer, and reference legs with SMA905 connectors for compatibility with most spectrometers, including Thorlabs' CMOS Spectrometers, and most light sources, including Thorlabs' Broadband Fiber-Coupled Light Sources. A sliding clamp at each Y-junction can be locked in place by tightening the 8-32 setscrew.
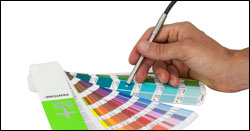
Click to Enlarge
RP26 Reflection Probe with Ø1/4" Probe Being Used to Make a Diffuse Reflection Color Measurement
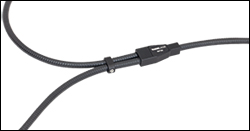
Click to Enlarge
The durable aluminum Y-joint of our bifurcated cables includes an adjustable fiber clamp, which is secured in place with an 8-32 locking screw.
Thorlabs also offers backscatter/reflection probes without the reference leg. These bundles are available with a sample end that terminates in either a Ø1/4" probe or an SMA905 connector. We also offer Ø1/4" probes with linear fiber bundle spectrometer ends; these provide increased spectrometer coupling efficiency for samples with low reflectance.
Reflection Probe Holders
Thorlabs offers fiber probe stands and holders (sold below), which secure a sample end probe in close proximity to a sample. They are easily adjustable and do not allow the fiber to move during measurements. Fiber optic probes can be secured with a vertical orientation for specular reflection spectroscopy or at 45° for diffuse reflection spectroscopy.
Bundles Selection Guide | |||||||||
---|---|---|---|---|---|---|---|---|---|
Straight | Bifurcated | Fan-Out | |||||||
![]() |
![]() |
![]() |
![]() |
![]() |
![]() |
![]() |
![]() |
![]() |
![]() |
Round | Linear to Linear |
Round to Linear |
Standard 2-Fiber Y-Bundle Optogenetics 2-Fiber Y-Bundle |
19-Fiber Y-Bundle |
Reflection Probes |
Reflection Probes with Reference Leg |
Transmission Dip Probes |
1-to-4 | Standard 1-to-7 Optogenetics 1-to-7 |
All Fiber Patch Cables |
Specifications | ||
---|---|---|
Wavelength Range | 250 - 1200 nma | 400 - 2400 nm |
Fiber Type | FG200UEA | FG200LEA |
Hydroxyl Ion Content | High OH | Low OH |
Fiber Core Size | Ø200 ± 4 µm | |
Fiber Cladding Diameter |
220 ± 2 µm | |
Fiber NA | 0.22 ± 0.02b | |
Fiber Attenuation Plot | ![]() |
|
Minimum Short Term Bend Radius | 23 mmc | |
Minimum Long Term Bend Radius | 46 mmc | |
Length | 2 +0.075/-0 m |
Reflection Spectroscopy Application
These reflection spectroscopy probes can be used along with our CMOS spectrometers, broadband fiber-coupled light sources, and the fiber probe holders (sold below) to take diffuse reflection, specular reflection, and color measurements while simultaneously monitoring the source. The red line on the computer below indicates the spectrum of the sample, while the blue line represents that of the source. This measurement was taken with two CCT11 spectrometers, an SLS201 tungsten-halogen stablized light source, the RP26 reflection probe bundle, and an RPS reflection probe stand. For more information about other items Thorlabs offers for use in such a setup (see below).
Spectrometers
Thorlabs offers several CMOS spectrometers for use in the visible, NIR, or UV to NIR spectral ranges. The CCT11 and CCT12 operate in the 350 - 700 nm and 500 - 1000 nm spectral ranges with <0.5 nm and <0.6 nm resolution, respectively. The extended-range CCT10 operates in the 200 - 1000 nm spectral range with <2.0 nm resolution, but the UV range may be heavily attenuated when analyzing broadband spectra.
Light Sources
The SLS201L tungsten-halogen broadband fiber-coupled light source delivers a 2796 K blackbody-type spectrum in the 360 - 2600 nm wavelength range and has active electronic stabilization for low spectral and intensity drift. Alternatively, the SLS203F Globar fiber-coupled light source provides a 1500 K temperature and 500 - 9000 nm emission range. We also offer fiber-coupled LEDs available with a selection of peak wavelengths or a broadband white-light emission spectra and our line of fiber-coupled laser sources offers a selection of options for intense single-wavelength illumination.
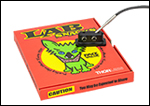
Click to Enlarge
Diffuse Measurement Taken at 45° Using the RPH Holder Block
Reflection Probe Fiber Bundles
The reflection probes offered on this page contain either high-OH or low-OH multimode fiber for wavelengths from 250 - 1200 nm and 400 - 2400 nm, respectively. These probes feature a sample end that terminates in a Ø1/4" probe. Thorlabs also offers reflection probes that do not have a reference leg. These probes are available with a sample end that terminates in either a Ø1/4" probe or an SMA905 connector. We also offer Ø1/4" and SMA-terminated probes with linear fiber bundle spectrometer ends, providing increased spectrometer coupling efficiency for samples with low reflectance.
If the coaxial illumination provided by a reflection probe bundle is not critical, separate fiber patch cables or bundles with SMA connectors can be used for illumination and signal collection. Our large-core round bundles maximize illumination intensity, while our single-fiber multimode SMA patch cables are useful for precise illumination or for connection to a fiber-coupled laser. We also offer round-to-linear fiber bundles, which maximize signal strength at the spectrometer.
Reflection Probe Holders
Thorlabs offers the RPS fiber probe stand (shown above and to the left), which allows for precise, stable positioning of the fiber optic probe at an angle of 90° or 45° relative to the sample. The probe holder arms (also sold separately) can also be integrated into other optomechanical setups using Ø1/2" posts. Alternatively, the RPH probe holder block sits directly on a sample, allowing the fiber tip to be positioned close to the surface and also blocking out room lights from the area under test.
Quick Links |
---|
Damage at the Air / Glass Interface |
Intrinsic Damage Threshold |
Preparation and Handling of Optical Fibers |
Laser-Induced Damage in Silica Optical Fibers
The following tutorial details damage mechanisms relevant to unterminated (bare) fiber, terminated optical fiber, and other fiber components from laser light sources. These mechanisms include damage that occurs at the air / glass interface (when free-space coupling or when using connectors) and in the optical fiber itself. A fiber component, such as a bare fiber, patch cable, or fused coupler, may have multiple potential avenues for damage (e.g., connectors, fiber end faces, and the device itself). The maximum power that a fiber can handle will always be limited by the lowest limit of any of these damage mechanisms.
While the damage threshold can be estimated using scaling relations and general rules, absolute damage thresholds in optical fibers are very application dependent and user specific. Users can use this guide to estimate a safe power level that minimizes the risk of damage. Following all appropriate preparation and handling guidelines, users should be able to operate a fiber component up to the specified maximum power level; if no maximum is specified for a component, users should abide by the "practical safe level" described below for safe operation of the component. Factors that can reduce power handling and cause damage to a fiber component include, but are not limited to, misalignment during fiber coupling, contamination of the fiber end face, or imperfections in the fiber itself. For further discussion about an optical fiber’s power handling abilities for a specific application, please contact Thorlabs’ Tech Support.
Damage at the Air / Glass Interface
There are several potential damage mechanisms that can occur at the air / glass interface. Light is incident on this interface when free-space coupling or when two fibers are mated using optical connectors. High-intensity light can damage the end face leading to reduced power handling and permanent damage to the fiber. For fibers terminated with optical connectors where the connectors are fixed to the fiber ends using epoxy, the heat generated by high-intensity light can burn the epoxy and leave residues on the fiber facet directly in the beam path.
Table 36C Estimated Optical Power Densities on Air / Glass Interfacea | ||
---|---|---|
Type | Theoretical Damage Thresholdb | Practical Safe Levelc |
CW (Average Power) |
~1 MW/cm2 | ~250 kW/cm2 |
10 ns Pulsed (Peak Power) |
~5 GW/cm2 | ~1 GW/cm2 |
Damage Mechanisms on the Bare Fiber End Face
Damage mechanisms on a fiber end face can be modeled similarly to bulk optics, and industry-standard damage thresholds for UV Fused Silica substrates can be applied to silica-based fiber. However, unlike bulk optics, the relevant surface areas and beam diameters involved at the air / glass interface of an optical fiber are very small, particularly for coupling into single mode (SM) fiber. therefore, for a given power density, the power incident on the fiber needs to be lower for a smaller beam diameter.
Table 36C lists two thresholds for optical power densities: a theoretical damage threshold and a "practical safe level". In general, the theoretical damage threshold represents the estimated maximum power density that can be incident on the fiber end face without risking damage with very good fiber end face and coupling conditions. The "practical safe level" power density represents minimal risk of fiber damage. Operating a fiber or component beyond the practical safe level is possible, but users must follow the appropriate handling instructions and verify performance at low powers prior to use.
Calculating the Effective Area for Single Mode Fibers
The effective area for single mode (SM) fiber is defined by the mode field diameter (MFD), which is the cross-sectional area through which light propagates in the fiber; this area includes the fiber core and also a portion of the cladding. To achieve good efficiency when coupling into a single mode fiber, the diameter of the input beam must match the MFD of the fiber.
As an example, SM400 single mode fiber has a mode field diameter (MFD) of ~Ø3 µm operating at 400 nm, while the MFD for SMF-28 Ultra single mode fiber operating at 1550 nm is Ø10.5 µm. The effective area for these fibers can be calculated as follows:
SM400 Fiber: Area = Pi x (MFD/2)2 = Pi x (1.5 µm)2 = 7.07 µm2 = 7.07 x 10-8 cm2
SMF-28 Ultra Fiber: Area = Pi x (MFD/2)2 = Pi x (5.25 µm)2 = 86.6 µm2 = 8.66 x 10-7 cm2
To estimate the power level that a fiber facet can handle, the power density is multiplied by the effective area. Please note that this calculation assumes a uniform intensity profile, but most laser beams exhibit a Gaussian-like shape within single mode fiber, resulting in a higher power density at the center of the beam compared to the edges. Therefore, these calculations will slightly overestimate the power corresponding to the damage threshold or the practical safe level. Using the estimated power densities assuming a CW light source, we can determine the corresponding power levels as:
SM400 Fiber: 7.07 x 10-8 cm2 x 1 MW/cm2 = 7.1 x 10-8 MW = 71 mW (Theoretical Damage Threshold)
7.07 x 10-8 cm2 x 250 kW/cm2 = 1.8 x 10-5 kW = 18 mW (Practical Safe Level)
SMF-28 Ultra Fiber: 8.66 x 10-7 cm2 x 1 MW/cm2 = 8.7 x 10-7 MW = 870 mW (Theoretical Damage Threshold)
8.66 x 10-7 cm2 x 250 kW/cm2 = 2.1 x 10-4 kW = 210 mW (Practical Safe Level)
Effective Area of Multimode Fibers
The effective area of a multimode (MM) fiber is defined by the core diameter, which is typically far larger than the MFD of an SM fiber. For optimal coupling, Thorlabs recommends focusing a beam to a spot roughly 70 - 80% of the core diameter. The larger effective area of MM fibers lowers the power density on the fiber end face, allowing higher optical powers (typically on the order of kilowatts) to be coupled into multimode fiber without damage.
Damage Mechanisms Related to Ferrule / Connector Termination
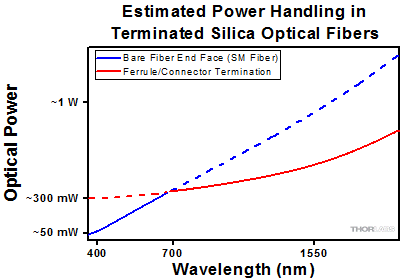
Figure 36D Plot showing approximate input power that can be incident on a single mode silica optical fiber with a termination. Each line shows the estimated power level due to a specific damage mechanism. The maximum power handling is limited by the lowest power level from all relevant damage mechanisms (indicated by a solid line).
Fibers terminated with optical connectors have additional power handling considerations. Fiber is typically terminated using epoxy to bond the fiber to a ceramic or steel ferrule. When light is coupled into the fiber through a connector, light that does not enter the core and propagate down the fiber is scattered into the outer layers of the fiber, into the ferrule, and the epoxy used to hold the fiber in the ferrule. If the light is intense enough, it can burn the epoxy, causing it to vaporize and deposit a residue on the face of the connector. This results in localized absorption sites on the fiber end face that reduce coupling efficiency and increase scattering, causing further damage.
For several reasons, epoxy-related damage is dependent on the wavelength. In general, light scatters more strongly at short wavelengths than at longer wavelengths. Misalignment when coupling is also more likely due to the small MFD of short-wavelength SM fiber that also produces more scattered light.
To minimize the risk of burning the epoxy, fiber connectors can be constructed to have an epoxy-free air gap between the optical fiber and ferrule near the fiber end face. Our high-power multimode fiber patch cables use connectors with this design feature.
Determining Power Handling with Multiple Damage Mechanisms
When fiber cables or components have multiple avenues for damage (e.g., fiber patch cables), the maximum power handling is always limited by the lowest damage threshold that is relevant to the fiber component. In general, this represents the highest input power that can be incident on the patch cable end face and not the coupled output power.
As an illustrative example, Figure 36D shows an estimate of the power handling limitations of a single mode fiber patch cable due to damage to the fiber end face and damage via an optical connector. The total input power handling of a terminated fiber at a given wavelength is limited by the lower of the two limitations at any given wavelength (indicated by the solid lines). A single mode fiber operating at around 488 nm is primarily limited by damage to the fiber end face (blue solid line), but fibers operating at 1550 nm are limited by damage to the optical connector (red solid line).
In the case of a multimode fiber, the effective mode area is defined by the core diameter, which is larger than the effective mode area for SM fiber. This results in a lower power density on the fiber end face and allows higher optical powers (on the order of kilowatts) to be coupled into the fiber without damage (not shown in graph). However, the damage limit of the ferrule / connector termination remains unchanged and as a result, the maximum power handling for a multimode fiber is limited by the ferrule and connector termination.
Please note that these are rough estimates of power levels where damage is very unlikely with proper handling and alignment procedures. It is worth noting that optical fibers are frequently used at power levels above those described here. However, these applications typically require expert users and testing at lower powers first to minimize risk of damage. Even still, optical fiber components should be considered a consumable lab supply if used at high power levels.
Intrinsic Damage Threshold
In addition to damage mechanisms at the air / glass interface, optical fibers also display power handling limitations due to damage mechanisms within the optical fiber itself. These limitations will affect all fiber components as they are intrinsic to the fiber itself. Two categories of damage within the fiber are damage from bend losses and damage from photodarkening.
Bend Losses
Bend losses occur when a fiber is bent to a point where light traveling in the core is incident on the core/cladding interface at an angle higher than the critical angle, making total internal reflection impossible. Under these circumstances, light escapes the fiber, often in a localized area. The light escaping the fiber typically has a high power density, which burns the fiber coating as well as any surrounding furcation tubing.
A special category of optical fiber, called double-clad fiber, can reduce the risk of bend-loss damage by allowing the fiber’s cladding (2nd layer) to also function as a waveguide in addition to the core. By making the critical angle of the cladding/coating interface higher than the critical angle of the core/clad interface, light that escapes the core is loosely confined within the cladding. It will then leak out over a distance of centimeters or meters instead of at one localized spot within the fiber, minimizing the risk of damage. Thorlabs manufactures and sells 0.22 NA double-clad multimode fiber, which boasts very high, megawatt range power handling.
Photodarkening
A second damage mechanism, called photodarkening or solarization, can occur in fibers used with ultraviolet or short-wavelength visible light, particularly those with germanium-doped cores. Fibers used at these wavelengths will experience increased attenuation over time. The mechanism that causes photodarkening is largely unknown, but several fiber designs have been developed to mitigate it. For example, fibers with a very low hydroxyl ion (OH) content have been found to resist photodarkening and using other dopants, such as fluorine, can also reduce photodarkening.
Even with the above strategies in place, all fibers eventually experience photodarkening when used with UV or short-wavelength light, and thus, fibers used at these wavelengths should be considered consumables.
Preparation and Handling of Optical Fibers
General Cleaning and Operation Guidelines
These general cleaning and operation guidelines are recommended for all fiber optic products. Users should still follow specific guidelines for an individual product as outlined in the support documentation or manual. Damage threshold calculations only apply when all appropriate cleaning and handling procedures are followed.
-
All light sources should be turned off prior to installing or integrating optical fibers (terminated or bare). This ensures that focused beams of light are not incident on fragile parts of the connector or fiber, which can possibly cause damage.
-
The power-handling capability of an optical fiber is directly linked to the quality of the fiber/connector end face. Always inspect the fiber end prior to connecting the fiber to an optical system. The fiber end face should be clean and clear of dirt and other contaminants that can cause scattering of coupled light. Bare fiber should be cleaved prior to use and users should inspect the fiber end to ensure a good quality cleave is achieved.
-
If an optical fiber is to be spliced into the optical system, users should first verify that the splice is of good quality at a low optical power prior to high-power use. Poor splice quality may increase light scattering at the splice interface, which can be a source of fiber damage.
-
Users should use low power when aligning the system and optimizing coupling; this minimizes exposure of other parts of the fiber (other than the core) to light. Damage from scattered light can occur if a high power beam is focused on the cladding, coating, or connector.
Tips for Using Fiber at Higher Optical Power
Optical fibers and fiber components should generally be operated within safe power level limits, but under ideal conditions (very good optical alignment and very clean optical end faces), the power handling of a fiber component may be increased. Users must verify the performance and stability of a fiber component within their system prior to increasing input or output power and follow all necessary safety and operation instructions. The tips below are useful suggestions when considering increasing optical power in an optical fiber or component.
-
Splicing a fiber component into a system using a fiber splicer can increase power handling as it minimizes possibility of air/fiber interface damage. Users should follow all appropriate guidelines to prepare and make a high-quality fiber splice. Poor splices can lead to scattering or regions of highly localized heat at the splice interface that can damage the fiber.
-
After connecting the fiber or component, the system should be tested and aligned using a light source at low power. The system power can be ramped up slowly to the desired output power while periodically verifying all components are properly aligned and that coupling efficiency is not changing with respect to optical launch power.
-
Bend losses that result from sharply bending a fiber can cause light to leak from the fiber in the stressed area. When operating at high power, the localized heating that can occur when a large amount of light escapes a small localized area (the stressed region) can damage the fiber. Avoid disturbing or accidently bending fibers during operation to minimize bend losses.
-
Users should always choose the appropriate optical fiber for a given application. For example, large-mode-area fibers are a good alternative to standard single mode fibers in high-power applications as they provide good beam quality with a larger MFD, decreasing the power density on the air/fiber interface.
-
Step-index silica single mode fibers are normally not used for ultraviolet light or high-peak-power pulsed applications due to the high spatial power densities associated with these applications.
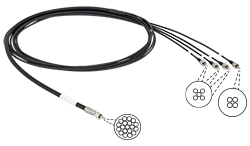
Click to Enlarge
Figure 31B Custom 1-to-4 Fan-Out Cable
Figure 31A Sample Fiber Bundle Connector Configurations
Custom Fiber Bundles
Thorlabs is pleased to offer custom straight and fan-out fiber bundles with random or mapped fiber configurations. Table 31D outlines some of our current bundle production capabilities. We are in the process of expanding these production capabilities, so do not hesitate to inquire if you do not see the bundle that you require described here.
Some custom bundles will require techniques outside of our usual production processes. As a result, we cannot guarantee that we will be able to make a bundle configuration to fit the requirements of your specific application. However, our engineers will be happy to work with you to determine if Thorlabs can produce a fiber bundle that fulfills your needs. To receive a quote, please provide a drawing or draft of your bundle configuration.
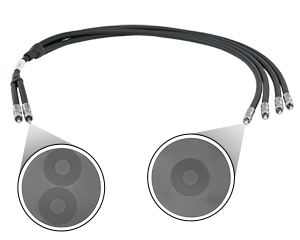
Click to Enlarge
Figure 31C Custom Silica Fiber Bundle with SMA905 Connectors
Table 31D Custom Bundle Capabilities | |||
---|---|---|---|
Bundle Configuration |
Straighta | Fan Out (2 or More Legs)a,b | |
Fiber Types |
Single Mode | Standard (320 to 2100 nm), Ultra-High NA (960 to 1600 nm), Photosensitive (980 to 1600 nm) |
|
Multimode | 0.10 NA Step Index (280 to 750 nm), 0.22 NA Step Index (190 to 2500 nm), 0.39 NA Step Index (300 to 2200 nm), Multimode Graded Index (750 to 1450 nm), Multimode ZrF4 (285 nm to 4.5 µm) |
||
Tubing Optionsc | Thorlabs' Stock Furcation Tubing, Stainless Steel Tubing or Black Heat Shrink Tubing | ||
Connectors | SMA905 (Ø2 mm Max Cored), FC/PC (Ø800 µm Max Cored), Ø1/4" Probe, or Flat-Cleaved Unterminated Fiber |
||
Length Tolerancee | ±0.14 m | ||
Active Area Geometryf |
Round or Linear | ||
Angle Polishing | On Special Request. Available for up to Ø105 µm Core on Single Fiber End. Please Inquire for More Information. |
Our cable engineers are available to help manufacture a bundle for your application.
Please contact techsales@thorlabs.com with your custom bundle requests.
Please provide a drawing or draft of your custom bundle to expedite quote processing.
Posted Comments: | |
No Comments Posted |

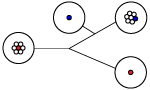
Click for Details
Fiber Configuration
Please note that the exact position of the fiber going to the reference leg is not guaranteed.
The RP26 and RP27 Reflection Probes feature SMA connectors on the light source, spectrometer, and reference legs and a Ø1/4" probe at the sample end. The light source leg consists of a 6 fiber bundle for maximizing light delivered to the sample, while the spectrometer and reference legs have a single fiber. The water-resistant Ø1/4" probe is 4.25" (10.8 cm) long and can be handheld or mounted using the RPS stand or RPH holder block (sold below). The light source, spectrometer, and reference ends are compatible with components that offer SMA bulkheads. For increased durability, these cables incorporate plastic-covered stainless steel protective tubing (FT061PS) and have reinforced metal strain relief boots.
Each patch cable includes four rubber protective caps that shield the connector ends from dust and other hazards. Additional CAPM Rubber Fiber Caps for SMA-terminated ends are also offered separately.
Item # | Hydroxyl Content |
Wavelength Range |
Fiber Item # |
Source Leg |
Sample Lega |
Spectrometer Leg |
Reference Leg |
Fiber Core Diameter |
Fiber Cladding Diameter |
NAb | Minimum Bend Radius | |
---|---|---|---|---|---|---|---|---|---|---|---|---|
Short Termc | Long Termc | |||||||||||
RP26 | High OH | 250 - 1200 nmd | FG200UEA | SMA, 7 Fibers | Ø1/4" Probe |
SMA, 1 Fiber | SMA, 1 Fiber | 200 ± 4 µm | 220 ± 2 µm | 0.22 ± 0.02 | 23 mm | 46 mm |
RP27 | Low OH | 400 - 2400 nm | FG200LEA |

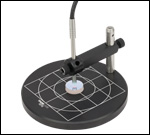
Click to Enlarge
Specular Reflectance Measurements at 90°
- Securely Hold Fiber Optic Probes with Ø1/4" Probe Sample Leg
- Orient Fiber Probe at 90° or 45° with Respect to the Sample
- Adjustable Height Arm Accommodates Samples up to at least 55 mm (2.16") Tall
- Ø6" (Ø152.4 mm) Base with Engraved Grid and Concentric Circles
- Replacement Arm Assembly Also Available
Thorlabs' RPS Adjustable Fiber Optic Probe Stand is designed to hold Ø1/4" fiber bundle probes above a sample at 45° for diffuse measurements or 90° for specular measurements. Each stand is comprised of an adjustable fiber holder arm (also available separately), a Ø1/2" optical post with engraved metric height scale, and a Ø6" (Ø152.4 mm) base with engraved concentric circles and grid pattern.
The Ø1/4" probe on the sample leg is held in place on the RPA arm using a TS25H thumbscrew. The arm's height can be adjusted using a TS25H thumbscrew, which has a spring-loaded, retractable Delrin®* tip. The spring-loaded tip provides sufficient force to hold the arm in place as final positional adjustments are being made, allowing for precise height adjustments. When using the RPS stand, samples up to 55 mm (2.16") tall can be accommodated using the included optical post. For taller samples, the included post can be easily replaced with a longer imperial or metric Ø1/2" post. The post is secured to the base by an M6 cap screw on the underside of the base, which can be removed using a 3/16" or 5 mm balldriver. If replacing the original post with an imperial Ø1/2" post, a SH25S063 1/4"-20 cap screw is also required.
Replacement RPA post holder arms are also available separately. These arms can also be used to mount Ø1/4" probes within custom optomechanical setups by securing them to Ø1/2" posts.
*Delrin® is a registered trademark of DuPont Polymers, Inc.

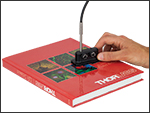
Click to Enlarge
Specular Reflectance Measurement Using an RPH Ø1/4" Probe Holder
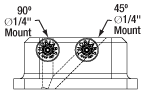
Click to Enlarge
Schematic of the RPH
- Secure Fiber Optic Probes at 90° or 45° with Respect to the Sample
- Take Reflectivity and Color Measurements of Flat Surfaces by Placing Holder Block Directly on Sample
The RPH Holder Block for Ø1/4" Fiber Optic Probes allows a fiber bundle tip to be placed directly above a sample. Background light may be blocked by covering the bore that is not in use with masking tape. Two ports are available for securing the probe at 45° or 90° with respect to the sample. The probe can be secured at various heights using one of two TS25H thumbscrews.
The 45° and the 90° ports share a common exit hole, as illustrated in the schematic of the RPH above.