Ytterbium Femtosecond Fiber Lasers, 1030 nm
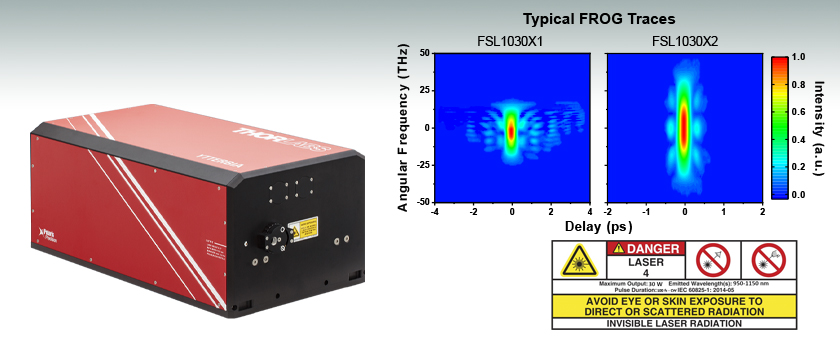
- User-Tunable Repetition Rate from 1 - 11 MHz
- Clean Ultrafast Pulses with Industry-Leading
Temporal Strehl Ratio of >0.85 - µJ-Class Pulse Energy
FSL1030X2
Ytterbium Femtosecond
Fiber Laser, 1030 nm

Please Wait
Key Specificationsa | |||
---|---|---|---|
Item # | FSL1030X1 | FSL1030X2 | |
Center Wavelength | 1030 ± 5 nm | ||
User Tunable Repetition Rateb | 1 - 11 MHz | ||
Pulse Width | Typical | <250 fs | <130 fs |
Max | <275 fs | <150 fs | |
Avg. Power at Max Rep Rateb, Min | >24 W | >20 W | |
Pulse Energyc, Max | 12 µJ | 2 µJ | |
Temporal Strehl Ratiod | Typical | >0.90 | |
Min | >0.85 |
Features
- Model for Higher Pulse Energy Applications
- FSL1030X1: 12 µJ, <250 fs (Typical)
- Model for Shorter Pulse Duration Applications
- FSL1030X2: 2 µJ, <130 fs (Typical)
- Ultrafast Pulse Width with Low Pulse Pedestal
- 1030 nm Center Wavelength
- User-Tunable Repetition Rate from 1 - 11 MHz
- >20 W Output Power at Max Rep Rate
- High Pulse Energy (See Table to the Right)
- Control via Downloadable Software for Hands-Free Operation (See Software Tab)
- Computer-Controlled Pulse Width Precompensation
- Compact Footprint: 569.0 mm x 320.0 mm x 237.7 mm
Applications
- Multiphoton Microscopy
- Photostimulated Optogenetics in Neuroscience
- Biological or Ophthalmic Tissue Machining
- Precision Micromachining of Tissues, Glass, and Plastics
- Optical Parametric Amplifier (OPA) Pumping
- White Light Supercontinuum Generation
- Non-Collinear Optical Parametric Amplifier (NOPA) Pumping
- Chemical Spectroscopy
- Terahertz Generation
Cascaded Domain Multiphoton Spatial Frequency Modulation Imaging
D. Scarbrough, A. Thomas, J. Field, R. Bartels, & J. Squier
Thorlabs' Ytterbia FSL1030X1 and FSL1030X2 Femtosecond Fiber Lasers are high peak power, NIR lasers that emit clean, ultrafast pulses centered at 1030 nm. With ultrafast pulse widths, pulse energies on the order of µJ, and a user-tunable repetition rate from 1 to 11 MHz, these fiber lasers enable a wide range of applications, including multiphoton microscopy, optogenetics, and precision machining, especially in ophthalmology. The high-energy pulses have typical temporal Strehl ratios of >0.90, corresponding to low temporal pedestals free from any picosecond background, thereby maximizing the usable output power per pulse. This is ideal for applications where reduced excitation powers are desirable to prevent heat-induced sample degradation and photobleaching, such as multiphoton stimulation in a neuroscience setting. Detailed specifications are available on the Specs tab.
A unique feature of the FSL1030X2 Ytterbia laser is the constant pulse energy as a function of repetition rate, which outputs 2 µJ pulses across the entire tunable repetition rate from 1 - 11 MHz. Alternatively, to achieve higher pulse energies, the FSL1030X1 Ytterbia laser has a constant pulse energy of 12 µJ from 1 - 2 MHz where it reaches a maximum average power of 24 W. At repetition rates above 2 MHz, the average power is maintained at 24 W, and the pulse energy decreases as the repetition rate increases. See the Specs tab for detailed specifications.
The key differences between the FSL1030X1 and FSL1030X2 laser systems are pulse energy and duration. The FSL1030X1 laser is ideal for applications where energy is the dominant requirement, such as nonlinear frequency conversion with an emphasis on net output. The FSL1030X2 laser is better suited for applications where reduced pulse duration is the main lever to improve the user’s signal level, such as ophthalmic tissue modification or multiphoton microscopy.
If your application requires higher pulse energies or shorter pulse widths, please contact LaserSales@thorlabs.com. An example of a custom pulse compression system is also described in the Application Note tab.
The Ytterbia fiber laser is designed for hands-free operation and long-term reliability. Parameters such as repetition rate, output power, and pulse duration can be controlled through a user-friendly GUI. When applying the computer-controlled pulse duration, pulse-width pre-compensation is accomplished without the use of external prisms or gratings. See the Software tab for details on the downloadable GUI.
Three CL5A mounting clamps are included with the laser head as well as two removable handles for easier set up. These lasers have a beam height of 120.7 mm (4.75"), marked on the side of the laser. Each laser also ships with a chiller and electronics unit, both of which are rack mountable.
Repetition Rate Tuning Details
The operation of the fiber laser is based upon an oscillator, pulse picker, and a chirped pulse amplifier, yielding reliable turn-key operation and exceptional long-term reliability. The pulse-picking system is based on frequency division of the femtosecond oscillator, which operates at 56 ± 2 MHz. The available repetition rates are given by this oscillator frequency divided by an integer from 5 to the value of the oscillator frequency. For example, when the oscillator frequency is 56 MHz, a divisor of 56 produces an amplified pulse train at 1 MHz, where 1 pulse out of every 56 is passed through the pulse picker and on to the amplifier. As the divisor becomes smaller, the spacing between accessible repetition rates becomes coarser. For example, for divisors 56 and 55, the resulting repetition rates are 1.00 and 1.02 MHz, respectively, while for divisors of 6 and 5, the repetition rates are 9.33 and 11.20 MHz, respectively. The maximum repetition rate for this example oscillator frequency is 11.2 MHz. The available repetition rates for which the laser is qualified are chosen from a drop-down menu in the software GUI.
Ytterbia Platform
The Ytterbia ultrafast laser platform utilizes a vertical stacking architecture, allowing additional modules, such as the Ytterbia Optical Parametric Amplifier (OPA), to be integrated into the same compact housing as the laser. In contrast to traditional wavelength conversion methods that require secondary enclosures and free space alignment, the Ytterbia platform offers enhanced alignment stability and opportunities for system reconfiguration. For more information about custom wavelength conversion modules available for use with the Ytterbia laser, please contact LaserSales@thorlabs.com.
Item # | FSL1030X1 | FSL1030X2 | ||
---|---|---|---|---|
Optical Specifications | ||||
Center Wavelength | 1030 ± 5 nm | |||
User Tunable Repetition Ratea | 1 - 11 MHz | |||
Pulse Duration (FWHM)b | Typical | <250 fs | <130 fs | |
Max | <275 fs | <150 fs | ||
Temporal Strehl Ratioc | Typical | >0.90 | ||
Min | >0.85 | |||
Pulse Energy, Maxd | 12 µJ | 2 µJ | ||
Average Power at Max Rep Rate, Min | >24 W | >20 W | ||
Beam Diameter (1/e2), Typical | 2.0 - 2.5 mm | |||
Mode Ellipticity | Typical | >0.9 | ||
Min | >0.8 | |||
Beam Quality (M2) | Typical | <1.15 | ||
Max | <1.2 | |||
Polarization | Linear, Vertical | |||
Polarization Extinction Ratio | >200:1 | |||
Power Stabilitye | <1% RMS Over 12 Hours | |||
Pointing Stabilitye, Typical | <10 μrad/°C | |||
Beam Height | 120.7 mm (4.75") | |||
Dispersion Compensation | -1 x 105 fs2 to 1 x 105 fs2 | |||
Optical Head Dimensions (L x W x H) | 569.0 mm x 320.0 mm x 237.7 mm (22.40" x 12.60" x 9.36") |
|||
Optical Head Weight | 36 kg (79 lbs) | |||
Electrical Requirements | ||||
Input Voltage | 100 - 240 V | |||
Frequency | 50 - 60 Hz | |||
Power Consumption, Max | Controller: 400 W Chiller: 600 W |
|||
Environmental Requirements | ||||
Room Temperature Range | 17 to 25 °C (63 to 77 °F) | |||
Room Temperature Stability | <3 °C (5.4 °F) Over 24 Hours |
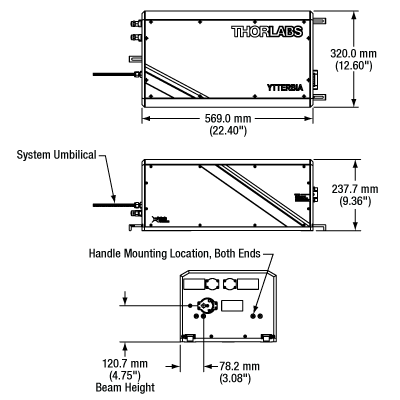
Click for Details
Laser Head Dimensions
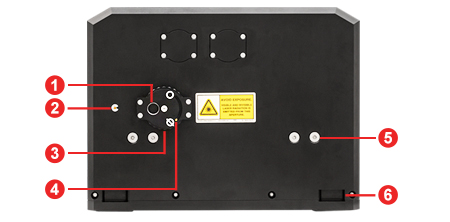
Click to Enlarge
Laser Head Front Panel
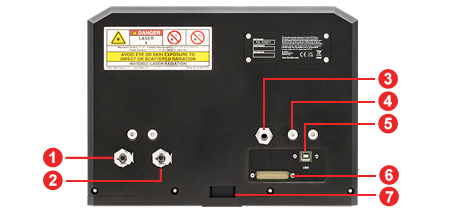
Click to Enlarge
Laser Head Back Panel
Front Panel | |
---|---|
Callout | Description |
1 | Laser Output Aperture |
2 | Emission Indicator |
3 | Manual Aperture Wheel to Open/Close Shutter |
4 | Aperture Shutter Position Indicator |
5 | Mounting Location for Removable Handles (4 Places) |
6 | Included CL5A Clamp Location (2 Places) |
Back Panel | |
---|---|
Callout | Description |
1 | Water In |
2 | Water Out |
3 | Fixed Optical Umbilical |
4 | Mounting Location for Removable Handles (4 Places) |
5 | USB 2.0 Type-B Connector |
6 | Sub-D 25 Pin Umbilical to Controller |
7 | Included CL5A Clamp Location |
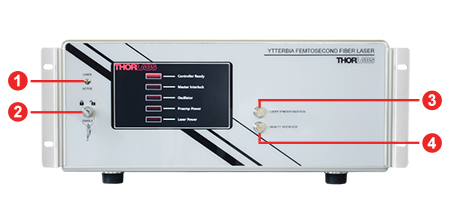
Click to Enlarge
Laser Controller Front Panel
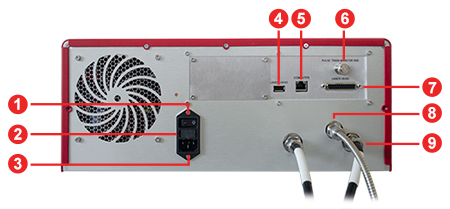
Click to Enlarge
Laser Controller Back Panel
Front Panel | |
---|---|
Callout | Description |
1 | Emission Indicator |
2 | Laser Enable Switch |
3 | Laser Synchronization Signal (BNC Female, TTL) |
4 | Remote Interlock (BNC Female, Open/Closed) |
Back Panel | |
---|---|
Callout | Description |
1 | AC Power On/Off Switch |
2 | Fuse Tray |
3 | AC Power Cord Connector |
4 | USB 2.0 Type A Connector to Laser |
5 | Ethernet Port to Computer |
6 | Oscillator Pulse Train Monitor (BNC Female, 50 Ω, <1 V) |
7 | Sub-D 25 Umbilical to Laser |
8 | Fixed Optical Umbilical to Laser |
9 | Fixed Water Lines (2 Places) |
The FSL1030X1 and FSL1030X2 femtosecond fiber lasers include the following components:
- Laser Head and Electronics Unit (Connected via Non-Detachable Umbilical, 2.5 m)
- Air-to-Water Chiller and Water Lines, with Valved Quick-Disconnect Fittings, 2.5 m
- Pre-Mixed Anti-Corrosion Fluid for the Chiller (Item # CDTX)
- Two Region-Specific Power Cords, 1.8 m
- Ethernet Cable, 2.5 m
- USB 2.0 Type-A to Type-B Cable, 2.5 m
- Ethernet to USB Converter
- Three CL5A Table Clamps for Mounting Laser
- Two Handles for Carrying
- USB Stick with Software and System Performance Data
An uninterruptable power supply (UPS) is available as an add-on to the laser purchase upon request.
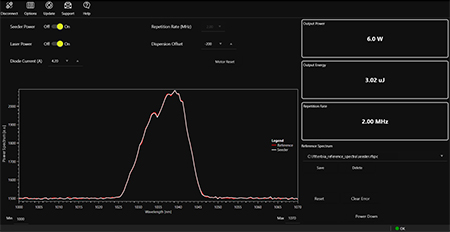
Click to Enlarge
Ytterbia Laser Software GUI
A Windows®-based software package is provided to give the user control of the laser while providing system feedback on the amplifier seed spectrum, system power levels, interlock status, and other diagnostics built into the laser system. More information on the software can be found in the manual.
Recommended System Requirements | |
---|---|
Operating System | Windows® 10 (64 Bit) |
Memory (RAM) | 5 GB |
Hard Drive | 30 MB (Min) of Available Disk Space |
Interface | Ethernet or USB 2.0 |
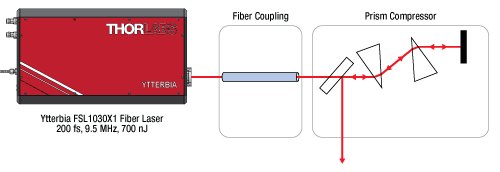
Click to Enlarge
Block Diagram of the Nonlinear Pulse Compression System
Custom Capability: Nonlinear Pulse Compression
The demand for ultrafast lasers has increasingly trended towards more power at shorter pulse durations while retaining ease of use, driven in part by the maturation of the many applications utilizing these ultrafast sources. Some example applications that stand to benefit from a reduction in pulse duration are biological material modification, micro-machining, time-resolved spectroscopy, and THz-generation. The system described here is available as a custom add-on for Ytterbia Series Fiber Lasers, providing pulses as short as 39 fs. This work was presented at the Conference on Lasers and Electro-Optics (CLEO) 2022.1
Ultrafast Ytterbium (Yb) fiber lasers in the near infrared combine high pulse energies and high repetition rates but generally have pulse durations in the few hundreds of femtoseconds due to limitations in gain-bandwidth and gain-narrowing. To overcome this limitation, spectral broadening techniques via self-phase modulation in a variety of optical systems and materials have been developed to shorten the pulse duration: single mode fiber at tens of nJ,2 specialty large-mode-area photonic crystal fiber at a few hundreds of nJ to a few µJ,3 gas-filled hollow core fiber at µJ to a few mJ,4 passage through multiple thin optical windows at µJ to a few mJ,5 and finally multi-pass cells with thin plates or high-pressure gas at mJ or higher energies.6
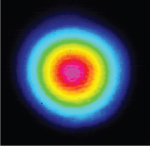
Click to Enlarge
The output collimated spatial mode of the multimode fiber shows the preservation of the launched LP0,1 mode while broadening the spectrum by a factor of 5.
Fiber-based systems are favorable in that the fundamental waveguide mode ensures a pristine output beam quality and high pointing stability. One of the main challenges to spectral broadening in any type of fiber is the potential for damage to the input fiber face, where at µJ-energy levels, self-focusing plays a critical role in limiting scalability. Spectral broadening solutions for pulse energies greater than 10’s of nJ require exotic or unattainable optical fibers, have catastrophic sensitivity to laser pointing, and introduce high system complexity and cost. To combat these difficulties, while retaining the advantages of a simple single mode fiber for nonlinear spectral broadening, we have pioneered an approach using the fundamental LP0,1 spatial mode of generic multimode fiber.
One example of the capabilities of this approach is presented here. A Thorlabs Ytterbia FSL1030X1 Yb fiber laser was coupled into a multimode fiber, <100 mm in length, with a calculated LP0,1 mode field diameter of ~65 µm. The 1035 nm, 9.5 MHz repetition rate, 200 fs input pulse was broadened to a spectral bandwidth supporting a 36 fs full-width-at-half-maximum (FWHM) transform-limited pulse duration at a pulse energy of 490 nJ (4.7 W of average power).
A Thorlabs' M² Measurement System confirmed a pristine spatial mode profile, with an M2 value of 1.05 and an ellipticity of 96%, as expected from waveguide-based spectral broadening (see the image of the spatial mode in the figure to the right). The output pulse from the prism compressor had a 39 fs FWHM and 10 MW of peak power, which is ~90% of the peak power fundamental limit. This pulse was characterized via second-harmonic generation frequency resolved optical gating (SHG-FROG).
The graphs below show the performance of the input pulse (top) and output pulse (bottom). For each set of graphs, the SHG-FROG measurement is presented on the left, with the reconstructed spectrum, phase presented in the middle. The transform-limited and reconstructed intensities are presented to the far right, with the peak power and pulse width labeled.
Critically, we have also confirmed that this method of spectral broadening is below the damage threshold of the employed fiber, having logged >1000 hours of runtime.
Please contact LaserSales@thorlabs.com to learn more about custom pulse compression systems.
FSL1030X1 Input Pulse |
![]() Click to Enlarge Input Second Harmonic Generation Frequency-Resolved Optical Gating (SHG-FROG) Measurement |
![]() Click to Enlarge Input Power Spectrum |
![]() Click to Enlarge Input Pulse Intensity |
Nonlinear Compressed Output Pulse |
![]() Click to Enlarge Output SHG-FROG Measurement |
![]() Click to Enlarge Output Power Spectrum |
![]() Click to Enlarge Output Pulse Intensity |
References
- L. Wooldridge, S. R. Domingue, M. S. Kirchner, and P. Fendel, "Degradation-Free Spectral Broadening in a Multimode Fiber with ~0.5 μJ Pulses," in Conference on Lasers and Electro-Optics, Optica Technical Digest (Optica Publishing Group, 2022), paper JW3A.51.
- S. R. Domingue and R. A. Bartels, "Nearly transform-limited sub-20-fs pulses at 1065 nm and >10 nJ enabled by a flat field ultrafast pulse shaper," Optics Letters. 2015; 40(2), 253-256.
- T. Eidam, F. Roser, O. Schmidt, J. Limpert, and A. Tunnermann, "57 w, 27 fs pulses from a fiber laser system using nonlinear compression," Applied Physics B: Lasers and Optics. 2008; 92, 9–12.
- T. Nagy et al, "Generation of three-cycle multi-millijoule laser pulses at 318 w average power," Optica. 2019; 6(11), 1423-1424.
- M. Seo, K. Tsendsuren, S. Mitra, M. Kling, and D. Kim, "High-contrast, intense single-cycle pulses from an all thin-solid-plate setup," Optics Letters. 2020; 45(2), 367-370.
- P. Rueda, F. Videla, T. Witting, G. A. Torchia, and F. J. Furch, "8 fs laser pulses from a compact gas-filled multi-pass cell," Optics Express. 2021; 29(17), 27004-27013.
Pulsed Laser Emission: Power and Energy Calculations
Determining whether emission from a pulsed laser is compatible with a device or application can require referencing parameters that are not supplied by the laser's manufacturer. When this is the case, the necessary parameters can typically be calculated from the available information. Calculating peak pulse power, average power, pulse energy, and related parameters can be necessary to achieve desired outcomes including:
- Protecting biological samples from harm.
- Measuring the pulsed laser emission without damaging photodetectors and other sensors.
- Exciting fluorescence and non-linear effects in materials.
Pulsed laser radiation parameters are illustrated in Figure 1 and described in the table. For quick reference, a list of equations is provided below. The document available for download provides this information, as well as an introduction to pulsed laser emission, an overview of relationships among the different parameters, and guidance for applying the calculations.
Equations: |
||||
![]() |
and | ![]() |
||
![]() |
||||
![]() |
||||
![]() |
||||
Peak power and average power calculated from each other: |
||||
![]() |
and | ![]() |
||
Peak power calculated from average power and duty cycle*: | ||||
![]() |
*Duty cycle (![]() |
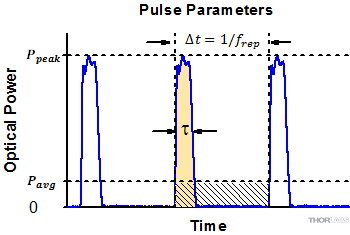
Click to Enlarge
Figure 1: Parameters used to describe pulsed laser emission are indicated in the plot (above) and described in the table (below). Pulse energy (E) is the shaded area under the pulse curve. Pulse energy is, equivalently, the area of the diagonally hashed region.
Parameter | Symbol | Units | Description | ||
---|---|---|---|---|---|
Pulse Energy | E | Joules [J] | A measure of one pulse's total emission, which is the only light emitted by the laser over the entire period. The pulse energy equals the shaded area, which is equivalent to the area covered by diagonal hash marks. | ||
Period | Δt | Seconds [s] | The amount of time between the start of one pulse and the start of the next. | ||
Average Power | Pavg | Watts [W] | The height on the optical power axis, if the energy emitted by the pulse were uniformly spread over the entire period. | ||
Instantaneous Power | P | Watts [W] | The optical power at a single, specific point in time. | ||
Peak Power | Ppeak | Watts [W] | The maximum instantaneous optical power output by the laser. | ||
Pulse Width | ![]() |
Seconds [s] | A measure of the time between the beginning and end of the pulse, typically based on the full width half maximum (FWHM) of the pulse shape. Also called pulse duration. | ||
Repetition Rate | frep | Hertz [Hz] | The frequency with which pulses are emitted. Equal to the reciprocal of the period. |
Example Calculation:
Is it safe to use a detector with a specified maximum peak optical input power of 75 mW to measure the following pulsed laser emission?
- Average Power: 1 mW
- Repetition Rate: 85 MHz
- Pulse Width: 10 fs
The energy per pulse:
seems low, but the peak pulse power is:
It is not safe to use the detector to measure this pulsed laser emission, since the peak power of the pulses is >5 orders of magnitude higher than the detector's maximum peak optical input power.
Laser Safety and Classification
Safe practices and proper usage of safety equipment should be taken into consideration when operating lasers. The eye is susceptible to injury, even from very low levels of laser light. Thorlabs offers a range of laser safety accessories that can be used to reduce the risk of accidents or injuries. Laser emission in the visible and near infrared spectral ranges has the greatest potential for retinal injury, as the cornea and lens are transparent to those wavelengths, and the lens can focus the laser energy onto the retina.
Safe Practices and Light Safety Accessories
- Laser safety eyewear must be worn whenever working with Class 3 or 4 lasers.
- Regardless of laser class, Thorlabs recommends the use of laser safety eyewear whenever working with laser beams with non-negligible powers, since metallic tools such as screwdrivers can accidentally redirect a beam.
- Laser goggles designed for specific wavelengths should be clearly available near laser setups to protect the wearer from unintentional laser reflections.
- Goggles are marked with the wavelength range over which protection is afforded and the minimum optical density within that range.
- Laser Safety Curtains and Laser Safety Fabric shield other parts of the lab from high energy lasers.
- Blackout Materials can prevent direct or reflected light from leaving the experimental setup area.
- Thorlabs' Enclosure Systems can be used to contain optical setups to isolate or minimize laser hazards.
- A fiber-pigtailed laser should always be turned off before connecting it to or disconnecting it from another fiber, especially when the laser is at power levels above 10 mW.
- All beams should be terminated at the edge of the table, and laboratory doors should be closed whenever a laser is in use.
- Do not place laser beams at eye level.
- Carry out experiments on an optical table such that all laser beams travel horizontally.
- Remove unnecessary reflective items such as reflective jewelry (e.g., rings, watches, etc.) while working near the beam path.
- Be aware that lenses and other optical devices may reflect a portion of the incident beam from the front or rear surface.
- Operate a laser at the minimum power necessary for any operation.
- If possible, reduce the output power of a laser during alignment procedures.
- Use beam shutters and filters to reduce the beam power.
- Post appropriate warning signs or labels near laser setups or rooms.
- Use a laser sign with a lightbox if operating Class 3R or 4 lasers (i.e., lasers requiring the use of a safety interlock).
- Do not use Laser Viewing Cards in place of a proper Beam Trap.
Laser Classification
Lasers are categorized into different classes according to their ability to cause eye and other damage. The International Electrotechnical Commission (IEC) is a global organization that prepares and publishes international standards for all electrical, electronic, and related technologies. The IEC document 60825-1 outlines the safety of laser products. A description of each class of laser is given below:
Class | Description | Warning Label |
---|---|---|
1 | This class of laser is safe under all conditions of normal use, including use with optical instruments for intrabeam viewing. Lasers in this class do not emit radiation at levels that may cause injury during normal operation, and therefore the maximum permissible exposure (MPE) cannot be exceeded. Class 1 lasers can also include enclosed, high-power lasers where exposure to the radiation is not possible without opening or shutting down the laser. | ![]() |
1M | Class 1M lasers are safe except when used in conjunction with optical components such as telescopes and microscopes. Lasers belonging to this class emit large-diameter or divergent beams, and the MPE cannot normally be exceeded unless focusing or imaging optics are used to narrow the beam. However, if the beam is refocused, the hazard may be increased and the class may be changed accordingly. | ![]() |
2 | Class 2 lasers, which are limited to 1 mW of visible continuous-wave radiation, are safe because the blink reflex will limit the exposure in the eye to 0.25 seconds. This category only applies to visible radiation (400 - 700 nm). | ![]() |
2M | Because of the blink reflex, this class of laser is classified as safe as long as the beam is not viewed through optical instruments. This laser class also applies to larger-diameter or diverging laser beams. | ![]() |
3R | Class 3R lasers produce visible and invisible light that is hazardous under direct and specular-reflection viewing conditions. Eye injuries may occur if you directly view the beam, especially when using optical instruments. Lasers in this class are considered safe as long as they are handled with restricted beam viewing. The MPE can be exceeded with this class of laser; however, this presents a low risk level to injury. Visible, continuous-wave lasers in this class are limited to 5 mW of output power. | ![]() |
3B | Class 3B lasers are hazardous to the eye if exposed directly. Diffuse reflections are usually not harmful, but may be when using higher-power Class 3B lasers. Safe handling of devices in this class includes wearing protective eyewear where direct viewing of the laser beam may occur. Lasers of this class must be equipped with a key switch and a safety interlock; moreover, laser safety signs should be used, such that the laser cannot be used without the safety light turning on. Laser products with power output near the upper range of Class 3B may also cause skin burns. | ![]() |
4 | This class of laser may cause damage to the skin, and also to the eye, even from the viewing of diffuse reflections. These hazards may also apply to indirect or non-specular reflections of the beam, even from apparently matte surfaces. Great care must be taken when handling these lasers. They also represent a fire risk, because they may ignite combustible material. Class 4 lasers must be equipped with a key switch and a safety interlock. | ![]() |
All class 2 lasers (and higher) must display, in addition to the corresponding sign above, this triangular warning sign. | ![]() |
Posted Comments: | |
Viktor Petrovsky
 (posted 2022-01-27 17:17:43.033) Please privide with price Femtosecond Ytterbium (Yb) Fiber Laser cdolbashian
 (posted 2022-01-31 05:18:45.0) Thank you for reaching out to us at Thorlabs. A member of our laser division will be in contact with you to discuss pricing and lead time. Artem Bogatyrev
 (posted 2021-12-06 09:00:30.91) Hello,
My university is looking for purchasing a laser. My department asks for a quote on this model: Thorlabs' Y-Fi™ Femtosecond Ytterbium Fiber, so that they can understand the standard pricing and potentially consider it for purchasing. Would you be so kind as to provide a quote for us?
The university is:
University of Nottingham
University Park
Nottingham, NG7 2RD
United Kingdom
Thank you, YLohia
 (posted 2021-12-23 12:17:33.0) Hello, quotes for this item should be requested by emailing LaserSales@thorlabs.com or your local Thorlabs Sales office. |